Problem
The production and operation of buildings represents one of the major sectors of global energy consumption, along with mobility, food, the production of consumer goods and electricity production. With the start of the industrial revolution, energy demand was increasingly met by fossil fuels, the combustion of which is associated with high greenhouse gas emissions and which – among other factors – contribute strongly to global warming. How can buildings be produced in the most energy-saving way possible, operated in an energy-efficient way and supplied with regenerative energies? What elementary planning principles need to be taken into account?
Generic Description
Energy-efficient building is a complex subject area that encompasses a target number of subsystems, design and construction patterns to be described in a separate “pattern catalogue”. At this point, only a few central planning principles for the energy-efficient operation of buildings can be presented. Otherwise, we refer to the extensive technical literature and results reports of research projects (e.g. Feist 1998, Voss et al. 2005, Hegger et al. 2007a, Hönger et al. 2009). When balancing building-related energy uses, a distinction must first be made between the production and operation of buildings. The energy used for the production and transport of building materials and components is referred to as grey energy (Spreng 1994) and can be determined through life cycle analyses. The production of many modern building materials such as concrete, steel, aluminium, glass and plastics is very energy-intensive. The use of renewable raw materials, such as wood, can reduce energy expenditure and improve the manufacturing balance.
With regard to the energy-efficient operation of buildings, a distinction must be made in the Central European climate between residential construction (“winter problem buildings”) and non-residential construction (“summer problem buildings”), for which different energy concepts and constructive, spatial and technical building measures are required due to divergent requirements. In residential construction, the greater share of energy demand is required for comfortable temperature control of the buildings in winter and for the seasonal transition periods. The focus is therefore on reducing heat losses, optimising passive solar energy use and efficient heat supply (Feist 1998, Hönger et al. 2009). The energy demand in non-residential buildings, on the other hand, is dominated by the energy demand for summer ventilation and cooling, artificial lighting and other use-specific energy demands (e.g. operation of IT servers) (Voss et al. 2005). Optimisation of daylight planning, good summer thermal insulation and concepts for largely natural ventilation play a major role here.
When designing energy-efficient buildings, the following general factors must be taken into account, prioritised here from passive to active elements (following Feist 1998):
- Target definitions on comfort criteria and construction methods resulting from the utilisation requirements and the space programme to be derived from them.
- Climate-friendly building: Consideration of macroclimatic (climate zone, seasonal temperatures, humidity, solar intensity) and microclimatic (wind protection, vegetation) boundary conditions.
- Compactness of buildings: Optimisation of the building typology and the surface-to-volume ratio (A/V ratio) in order to minimise the geometrically induced heat loss of buildings.
- Optimisation of the energy quality of the envelope surfaces: Constructions with low U-values for winter thermal insulation, structural and technical sun protection devices for summer thermal insulation, optimisation of the area ratio and orientation of window surfaces, avoidance of thermal bridges.
- Optimisation of building dynamics: good storage capacity of interior components to make good use of solar heat gains.
- Optimisation of building services systems for heating, ventilation and, if necessary, cooling.
- Integration of systems for the generation and storage of renewable energies, such as solar collectors, photovoltaics, storage systems.
- Adapt user behaviour and avoid rebound effects: The users of buildings need a basic understanding of energy processes so that their behaviour does not counteract the intended energy savings.
Taking these principles into account, there are various planning approaches to achieve the goal of energy-efficient buildings. Since the 1990s, passive house construction and zero or plus energy buildings based on it have established themselves as a widespread standard. The term passive house describes a building standard that can be realised with different construction methods, building forms and building materials. It is a further development of the low-energy house (NEH) standard and characterises buildings in which a comfortable indoor climate can be guaranteed in summer and winter without a separate heating or cooling system (Krapmeier & Drössler 2001). The limit values are a heating requirement of max. 15 kWh/m² living space and year and a primary energy requirement of max. 40 kWh/m2 living space and year. The basic principles of a passive house are (1) minimisation of heat loss, (2) controlled ventilation with heat recovery and (3) optimisation of solar gains. The prerequisite for a passive house is that the necessary amount of fresh air is able to transport the required heat for the maximum heating output. For the building, this means that energy losses must not exceed 10W/m² of living space. The first Passive House was built in Darmstadt-Kranichstein in 1991, at the same time as the energy-autonomous Solar House in Freiburg.
Based on the experience of Passive House construction and research on an energy-autonomous solar house (Voss 1997) as well as practice-oriented settlement concepts (Disch 2010) and other scientific studies on climate-neutral buildings (Musall 2015), the term zero- or plus-energy building has become established. These are mostly passive houses that generate as much or even more energy per year as they need for their operation through building-integrated systems for the generation of renewable energy.
In addition, there are many other planning approaches, such as building cybernetics (e.g. Pfeifer 2002, Tersluisen 2009), low-exergy (e.g. Leibundgut 2007), low-tech construction methods (e.g. Nagler et al. 2019) and combinations of these approaches (see e.g. 2226 by Baumschlager Eberle, in: Schoof 2014 and Aicher 2021), which can only be referred to here (Prytula 2011, pp.81-92).
Example
The three buildings of the Spreefeld have a minimised structural system of reinforced concrete with an outer shell of a non-bearing, prefabricated timber panel construction with cellulose insulation. With a U-value of 0.095 [W/m²K], this is highly insulating and suitable for passive houses (Carpaneto et al. 2011). On the parts where the reinforced concrete is on the outside, a thermal insulation composite system and mineral plaster was used to insulate, U-value of 0.184 [W/m²K]. The windows and balcony doors are made of wooden frames with triple insulating glazing and have a U-value of 0.86 [W/m²K]. The total area of the windows is approx. 43%, the south-east and south-west facing windows have external sun protection. The balconies, made of precast concrete slabs, are thermally separated from the floor slabs.
Heat is supplied from a central heating system with a natural gas-fired combined heat and power unit. The hot water supply is supported by solar thermal energy and electric instantaneous water heaters. The entire roof surfaces are equipped with photovoltaic systems. Decentralised ventilation systems provide supply and exhaust air with heat recovery. The compact architecture allows for a space- and energy-saving construction method, and through slow, user-adapted planning, particularly low communal production costs were realised. Recycling-relevant information on the materials used is available to the residents. As few materials as possible with admixtures were used. A small proportion of the exterior walls and insulation can be composted (Plaga et al. 2021).
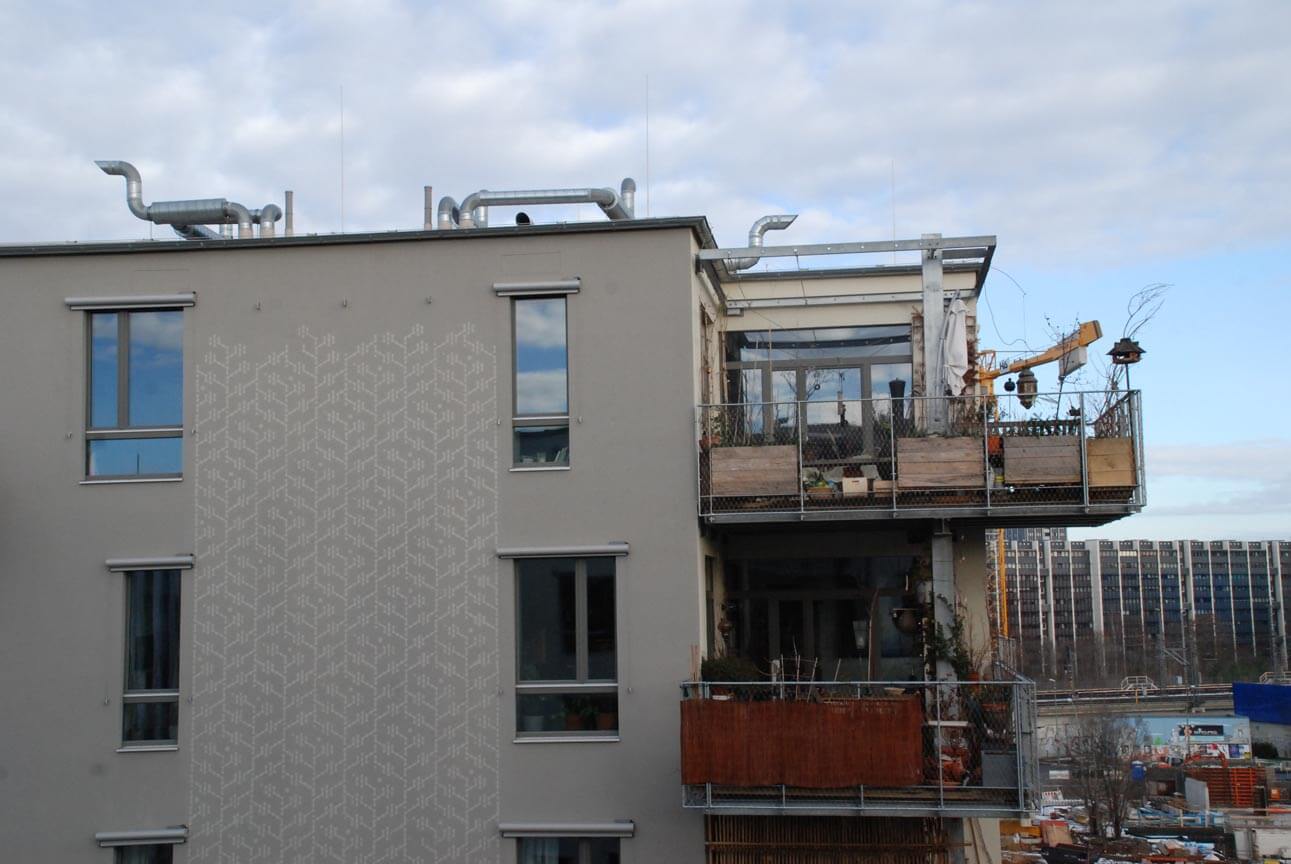
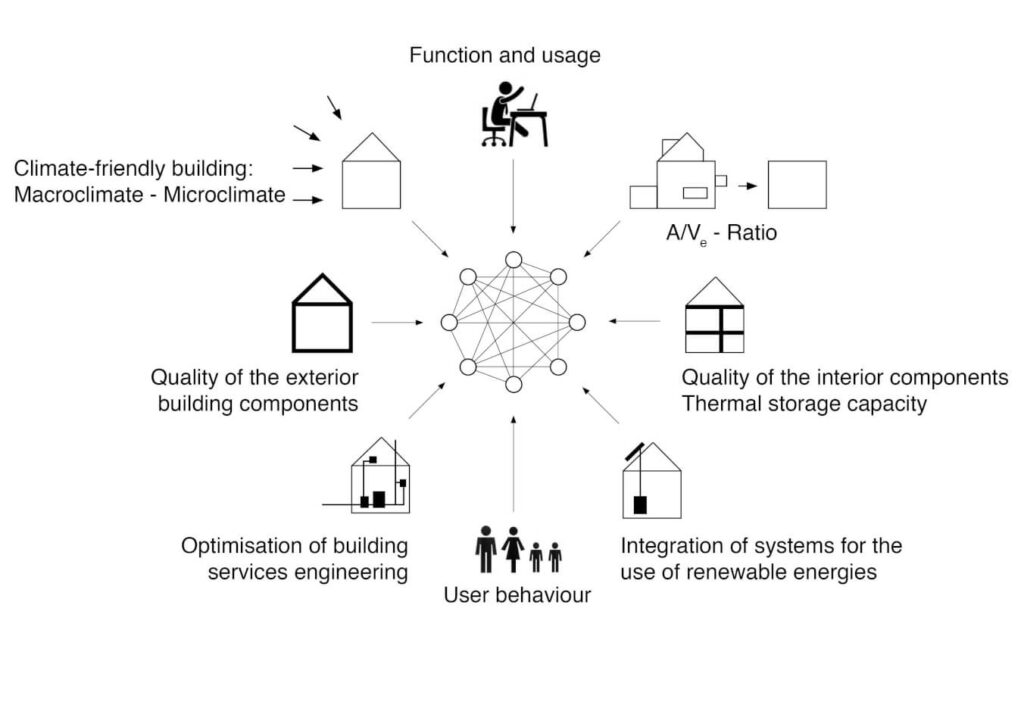
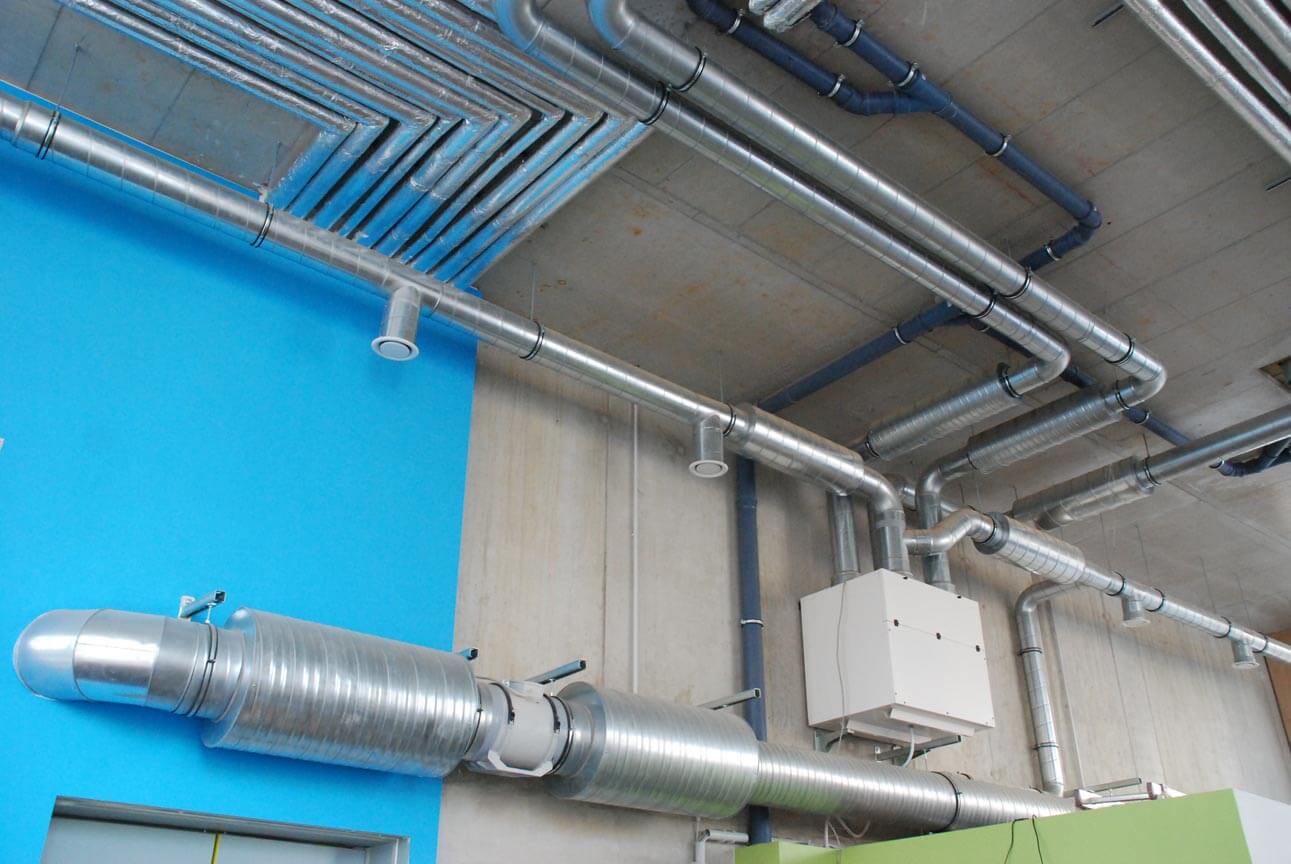
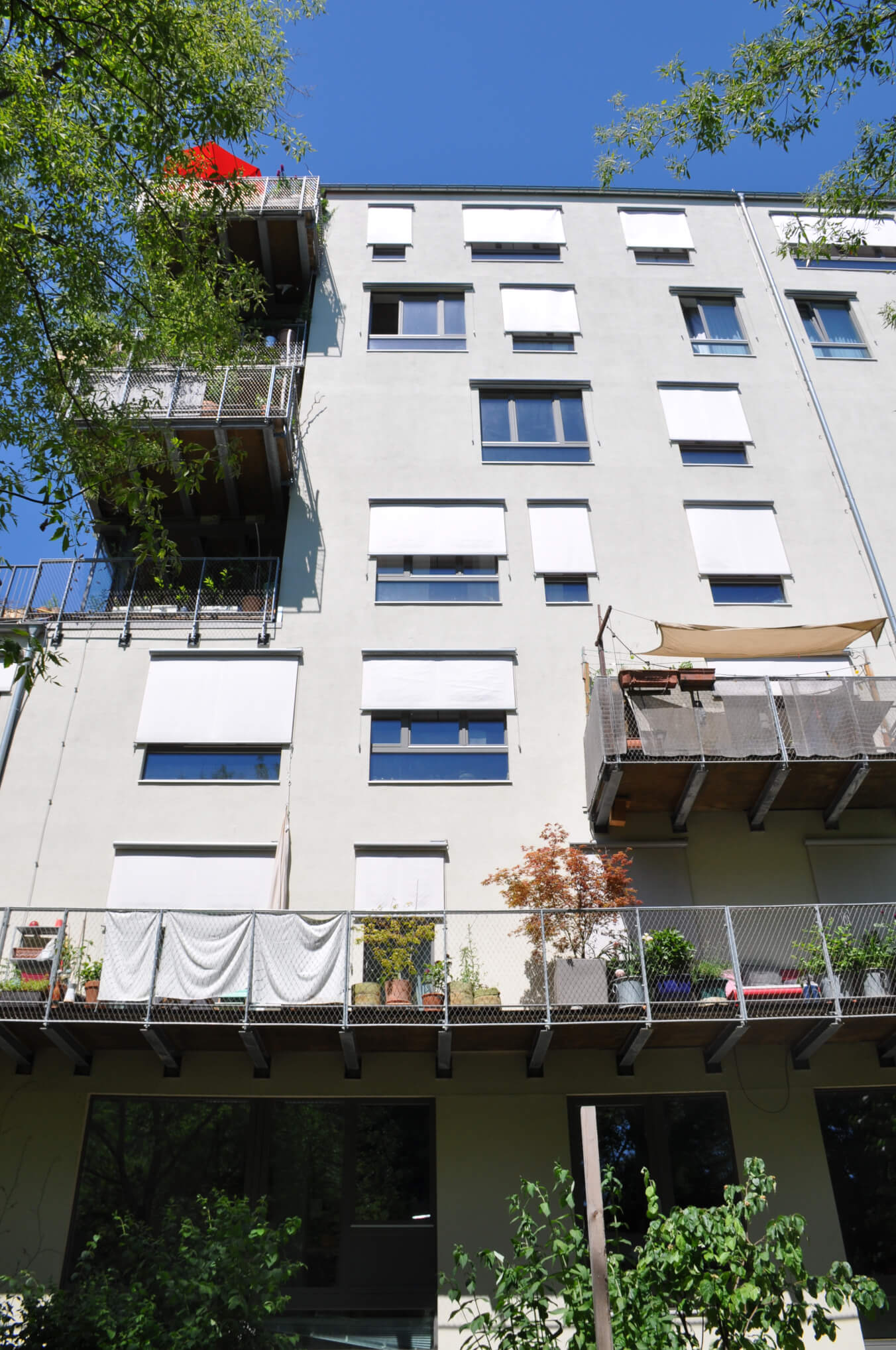
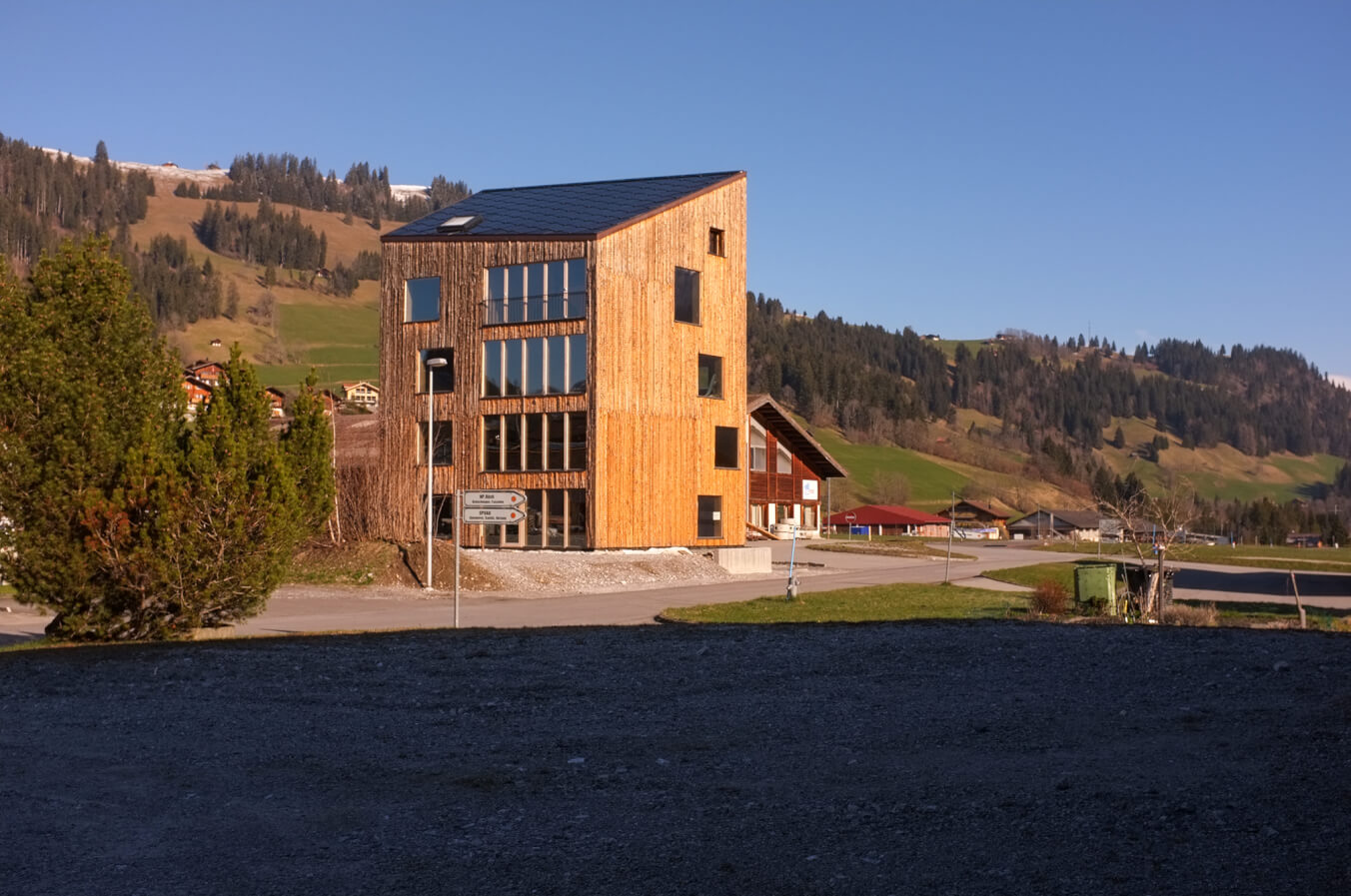
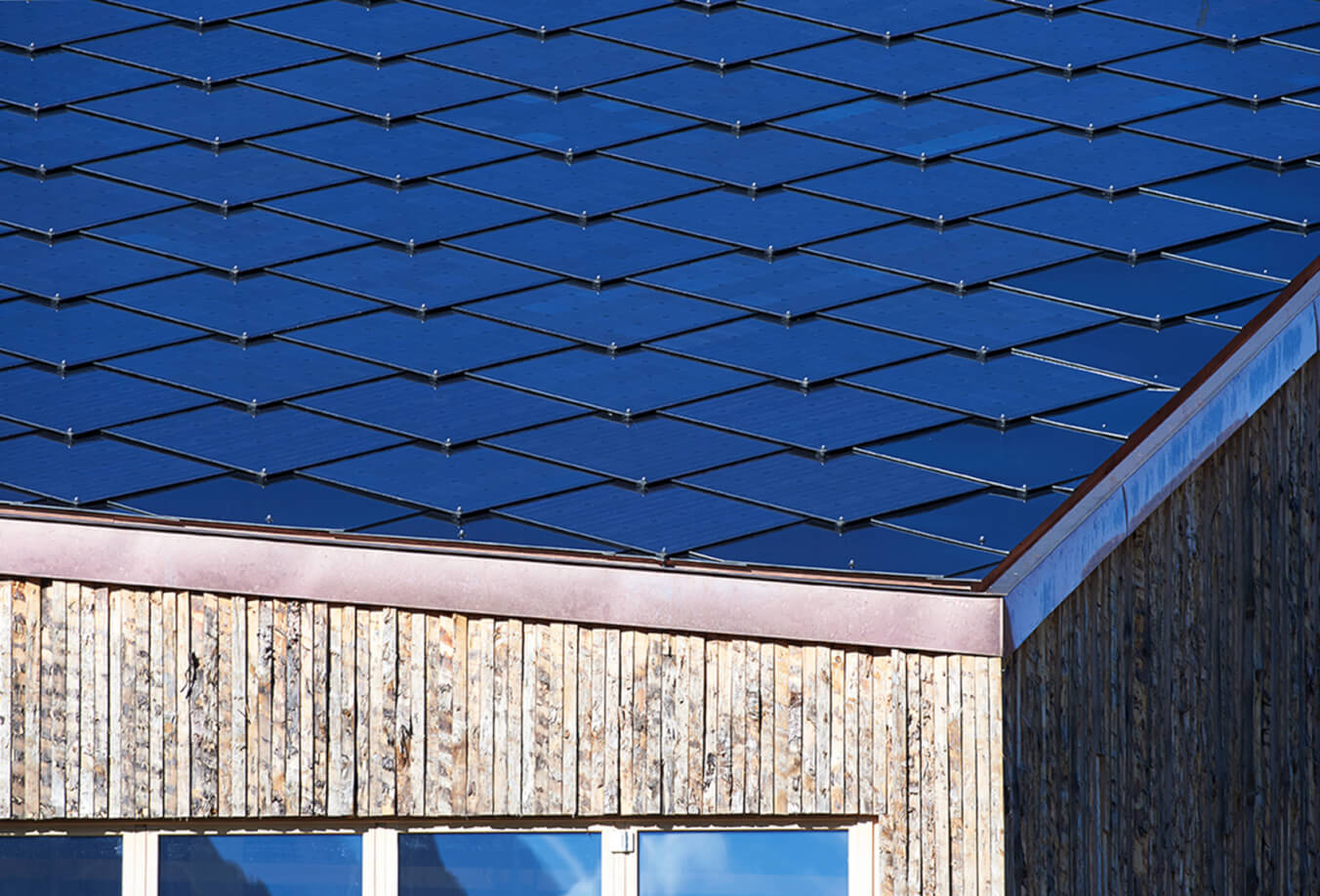
Lessons learned and synergies
In the planning and realisation of energy-efficient buildings, great progress has been made in the last 35-40 years through research, technological developments, new simulation methods and suitable legal and economic framework conditions. In order to achieve the overarching goal of a climate-neutral and sustainable heat supply, various planning and construction strategies can be used, such as passive house, zero and plus energy building, building cybernetics, low energy. Each of these sets different priorities with regard to the spatial-constructional, constructional or building-technical aspects. In the last few years, strategies for sector coupling have also been developed (waste heat recovery from waste or grey water, alternating storage use for electromobility and building technology), which not only shift the spatial balance limits.
It is known from many research projects that user behaviour plays a major role in actual energy consumption. The conceptual and technological robustness of the chosen measures therefore plays a major role, as does the knowledge of rebound effects, for example when the heated floor space or indoor air temperatures increase in energy-efficient buildings, and efficiency gains are thus cancelled out. Last but not least, it must be taken into account that energy-efficient building is only one subsystem of sustainable building and must always be considered in connection with other sustainability goals and protected goods (Hegger et al. 2007a, 2007b). However, energy-efficient buildings are an indispensable component of strategies for realising an energy-efficient and climate-neutral city, such as the 2000-watt society.
Sources
Aicher, Florian (2021): 2226 revisited. In: Bauwelt 22.2021, S. 36-43. Accessed on 10.05.2022 from https://www.2226.eu/fileadmin/user_upload/aktuelles/Home_Aktuelles/Publikationen/20211029_2006_BUR_LUS_6202_2226_Lustenau_Clipping_Print_Bauwelt_Heft_22_DE.pdf
Carpaneto, Silvia et al. (2011): Baubeschreibung zur Genehmigungsplanung, Stand 16.12.2011. Silvia Carpaneto – FAT KOEHL- BAR architekten gemeinschaft, Berlin
Disch, Rolf (2010: Häuser als Kraftwerke. Neue Siedlungen von Rolf Disch SolarArchitektur. Das Prinzip Plusenergie. Accessed on 10.05.2022 from http://www.rolfdisch.de/wp-content/uploads/BROSCHUERE_HA%CC%88USER_ALS_KRAFTWERKEEINZELSEITENSEITEN-1.pdf
Feist, Wolfgang (1998): Das Niedrigenergiehaus. C.F. Müller Verlag, Heidelberg
Hegger, Manfred et al. (2007 a): Energie Atlas: Nachhaltige Architektur (Detail Atlas). Autoren: Manfred Hegger, Matthias Fuchs, Thomas Stark, Martin Zeumer. Birkhäuser Verlag AG: Basel
Hegger, Manfred et al. (2007 b): Bewertung der Nachhaltigkeit von Gebäuden anhand von 20 Beispielprojekten als konkrete Handlungslinie und Arbeitshilfe für Planer. Abschlussbericht des Forschungsvorhabens gefördert unter AZ 24084-25 durch die Deutsche Bundesstiftung Umwelt (DBU). Autoren: Manfred Hegger, Matthias Fuchs, Thomas Stark, Martin Zeumer. Accessed on 12.05.2022 from https://www.dbu.de/OPAC/ab/DBU-Abschlussbericht-AZ-24084.pdf
Hönger, Christian et al. (2009): Das Klima als Entwurfsfaktor / Climate as a design factor. 1. Band der Reihe Laboratorium, Hochschule Luzern, Autoren: Christian Hönger, Roman Brunner, Urs-Peter Menti, Christoph Wieser. Quart Verlag, Luzern
Krapmeier, Helmut; Drössler, Eckart (2001): Cepheus – Wohnkomfort ohne Heizung. Schlussdokument des Projektes Cepheus Austria 1998-2001 – Wien: Springer
Leibundgut, Hansjürg (2007): viaGialla. Wegbeschreibung für Gebäude in eine nachhaltige Energie-Zukunft. Version 2.1
Musall, Eike (2015): Klimaneutrale Gebäude – Internationale Konzepte, Umsetzungsstrategien und Bewertungsverfahren für Null- und Plusenergiegebäude. Vollständiger Abdruck der von der Fakultät für Architektur und Bauingenieurwesen der Bergischen Universität Wuppertal genehmigten Dissertation. Accessed on 10.05.2022 from http://elpub.bib.uni-wuppertal.de/edocs/dokumente/fbd/architektur/diss2015/musall/dd1507.pdf
Nagler, Florian et al. (2019): Einfach Bauen. Endbericht des Forschungsvorhabens “Einfach Bauen. Ganzheitliche Strategien für energieeffizientes, einfaches Bauen – Untersuchung der Wechselwirkung von Raum, Technik, Material und Konstruktion” gefördert vom BBSR Zukunft Bau, Förderkennzeichen SWD-10.08.18.7-16.29. Autoren: Florian Nagler, Tilmann Jarmer, Anne Niemann, Antonia Cruel, Thomas Auer, Laura Franke, Hermann Kaufmann, Stefan Winter, Stephan Ott, Marco Krechel, Christoph Gehlen, Charlotte Thiel. Fraunhofer IRB Verlag. Accessed on 10.05.2022 from https://www.einfach-bauen.net/wp-content/uploads/2019/04/einfach-bauen-schlussbericht.pdf
Pfeifer, Günter (2002): Das kybernetische Prinzip. in: Der Architekt 11/2002, 37-44
Plaga, Tim et al. (2021): Projektdokumentation Spreefeld. Seminar Theorie und Praxis sozial-ökologischer Modellprojekte im WIntersemester 2020/21. Autoren: Tim Plaga, Johannes Popp, Nioosha Ravanshadi, Katharina Schürmann, Ulrike Silz, Lajana Tiedke. Fachhochschule Potsdam
Prytula, M. (2011): Ein integrales Energie- und Stoffstrommodell als Grundlage zur Bewertung einer nachhaltigen Entwicklung urbaner Systeme. Dissertation an der TU Berlin, Universitätsverlag der TU Berlin. Accessed on 10.05.2022 from https://depositonce.tu-berlin.de/handle/11303/3425
Schoof, Jakob (2014): Haus ohne Heizung: Bürogebäude von Baumschlager Eberle in Lustenau. In: Detail. Accessed on 10.05.2022 from https://www.detail.de/de/de_de/haus-ohne-heizung-buerogebaeude-von-baumschlager-eberle-in-lustenau-11703
Spreng, Daniel (1994): Graue Energie, Hochschulverlag Zürich / B.G. Teubner Stuttgart/Leipzig
Tersluisen, Angèle (2009): Effizienz als Prinzip. Planungstools für klimagerechtes Bauen. in: Der Architekt 03/2009: Ästhetik der Ökologie, Aufbruch in die klimatische Moderne, wiederveröffentlicht 04.04.2019. Accessed on 10.05.2022 from http://derarchitektbda.de/effizienz-als-prinzip/
Voss, Karsten (Hrsg.) (1997): Konzeption und Bau eines energieautarken Solarhauses Schlussbericht. – Stuttgart: Fraunhofer IRB Verlag
Voss, Karsten et al. (2005): Bürogebäude mit Zukunft. Konzepte, Analysen, Erfahrungen. Autoren: Karsten Voss, Günther Löhnert, Sebastian Herkel, Andreas Wagner, Matthias Wambsganß (Hrsg.). Berlin: Solarpraxis GmbH.